Abbreviations:
CCGT: combined-cycle gas turbine, this is the top-of-the-range gas turbine
CCS: Carbon Capture and Storage
GENCOST: Gencost
GW: gigawatt, 1,000 billion watts
IEA: International Energy Agency
NZ2050: Net Zero by 2050
PV: photovoltaic
RPV: reactor pressure vessel
UNECE: United Nations Economic Commission for Europe
Nuclear construction costs
In Part 1 of this series, we saw a group of US renewable researchers and noted that they accept that total electricity grid costs can be reduced by adding reliable sources; biomass, nuclear, gas with Carbon Capture and Storage (CCS), or hydro. But what about the construction and operating costs of nuclear power considered in isolation?
The plan for this section, is firstly to try to understand what determines the cost of building nuclear power plants. Is it the sheer volume of steel and concrete? Is it the amount of rare and precious materials? Is it the complexity of the construction? Is the industry overregulated? Is it simply price gauging? Is it the price of money coupled with long construction times?
Some of that will require considering the reasons for regulation. Is it because of accident risks? Or other presumptive risks, like nuclear terrorism?
In short, we’ll explore what the cheapest cost of reactors might be if we only had to pay for the materials and construction in what I’d call a rational regulatory environment.
Why consider theoretically lower costs?
Why bother considering costs in a non-existent rational regulatory regime? Because many people, particularly in Australia, are betting on nuclear being outrageously expensive. This is already false in the countries which supply most of our phones and some of our cars – China and South Korea. Even modest reform of nuclear regulatory regimes could dramatically reduce the costs of nuclear construction.
If nuclear isn’t outrageously expensive, then nobody will bother building a fleet of supertankers to ship hydrogen thousands of kilometres between continents. This will destroy any hopes some in Australia might have of being a hydrogen superpower.
If nuclear isn’t outrageously expensive, users of hydrogen will do what they do now, make it close to where it is needed, but using nuclear power instead of natural gas.
Countries which have recently announced substantial nuclear plans include :
France — perhaps 14 new large reactors by 2050;
Japan — reopening reactors;
Poland — 6-9 large reactors by 2040;
China — China is building and testing almost every kind of reactor anybody has imagined: fast neutron reactors that can run on nuclear waste from current reactors, high-temperature reactors that are perfect for making very cheap hydrogen, low-temperature reactors perfect for district heating, SMRs which can be built underground in clusters;
Indonesia — multiple activities in both large and smaller reactors;
South Korea — reverse previous Government policy and support nuclear both in Korea and as a major export industry;
Netherlands — Rolls-Royce to export SMR technology;
Turkey — 4 reactors nearing completion, another 4 planned;
Hungary — two 1.2 GW Russian reactors; these are unaffected by Russian sanctions;
the US — The US situation is complex. The recent taxation changes in the Inflation Reduction Act put nuclear on a more even playing field with renewables; one nuclear watcher thinks this could drive the construction of 300 (smallish) reactors over the next 30 years. The recent approval of the NuScale design may indicate a shift in the regulatory regime, or perhaps it was just a fluke.
If the result of all this activity is just one good, cheap, small modular reactor, then you can kiss goodbye to any thoughts of Australia being a green energy superpower. We won’t just miss the green energy superpower bus, but the green steel and aluminium bus, and the green concrete bus. We will continue to be a country whose prosperity depends on the prices we get for digging things up and selling them.
Theoretical future nuclear costs based on engineering, not regulation
When you look at the tiny amounts of stuff that need to be mined and fabricated to build and run a nuclear reactor, compared to wind and solar farms, you have to wonder why reactors are expensive.
A recent United Nations Economic Commission for Europe (UNECE) comparison of current nuclear plants with other electricity generation technology for selected valuable mineral resources came up with the graph in Figure 1. which compares technologies by the amounts of zinc, silicon, nickel, molybdenum, manganese, copper, cobalt, chromium and aluminium they require.
Recall, of course, that photovoltaic (PV) panels and wind turbines can’t really be compared to nuclear plants without adding in the batteries and extra transmission lines required to plug intermittency holes. So the bars for these technologies should be significantly higher, and higher again if we added in Lithium. We covered this in Part I; comparing nuclear with renewables is like comparing a balanced diet with boxes of donuts.

As you can see from the table, nuclear uses far less of all the chosen elements, so what is it that bumps the price of reactors up? It isn’t uranium. Fuel for reactors has nothing to do with construction costs and is typically around 0.5 cents/kilowatt hour during operation.
Case study: Comparing PV with nuclear
Check any solar PV panel specification sheet and scale it up, and you’ll find that it takes between 60,000 and 100,000 tonnes of stuff to make a GW of panels, and plenty of that stuff is fairly exotic as we have just seen.
Globally, about 70% of PV panels are in solar farms, with only about 13% on residential rooftops. Note that solar farms tend to use heavier more robust panels than residential systems. Mounting panels in a solar farm will require an additional similar tonnage of stuff – typically, aluminium and steel.
Next, you have to factor in that you need about 4-5 GW of solar PV to generate as much electricity annually as 1 GW of nuclear power. So, very roughly you can multiply your 'stuff' figure by 8 to 10, meaning you need 480,000 to 1,000,000 tonnes of stuff to get the same electricity as a 1 GW reactor would deliver in a year.
And even when you have your 4-5 GW of solar, you still need to factor in batteries to make a remotely equitable comparison.
What do you need to make a nuclear reactor?
Figure 2 is a schematic for a nuclear power plant.
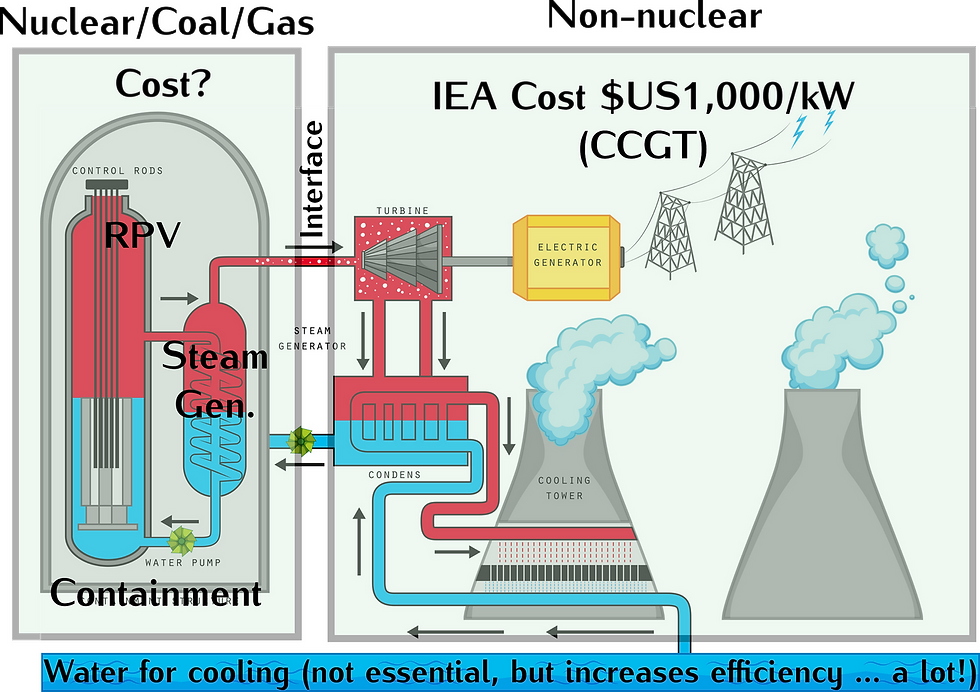
The nuclear bit is on the left and the non-nuclear bit is on the right. The stuff on the left is the reactor pressure vessel (RPV), a steam generator, and a big concrete building (called the containment) to protect them from large flying objects, e.g., planes. Really. Had the 9/11 hijackers chosen to fly into a nuclear reactor, then, apart from those on board obviously, and workers at the reactor, nobody would have died. Here is a clip showing a fighter jet being run into a simulated reactor building (a slab of very reinforced concrete). It made a dent about 60mm deep. A fully loaded passenger plane will do less damage; it will just crumple. You’d cause maximum damage by aiming your wing engine at the reactor. But that’s irrelevant – the plane will lose. I suspect the 9/11 hijackers knew this; perhaps not.
Suppose you bury the reactor? Bury the reactor and you need far less concrete. Earth can make a perfectly reasonable and much cheaper containment structure; your concrete and steel needs will plummet. Many SMR designs are taking this route.
If you look inside the concrete building and consider, for example, a 1.4GW RPV for a South Korean APR1400 reactor, it weighs just 573 tonnes; it’s about 15m high and 6m in diameter. As steel goes, it’s a bit special, but not spectacularly so. Welding 200mm thick steel sections for things like this used to take months, but a recent breakthrough allows it to be done in hours.
In this particular schematic, the steam generators are separate from, but close to the reactor, and inside the concrete containment building. That’s pretty normal, but not essential. Some SMR designs integrate the steam generation with the RPV.
Besides the RPV and its containment building, the remainder of the functional stuff, the stuff on the right of the schematic, is turbines, electrical generators and pipes ... lots of pipes. None of this stuff is nuclear stuff. All of this stuff should just be a few thousand tonnes of the same off-the-shelf gear you’d use in a coal plant. Except that the nuclear industry is very much like Apple. They like all their stuff to be different from everybody else’s stuff so they can gouge the hell out of you for every trivial little part. But that’s not really true or fair. There is a long history of US regulators driving up complexity to drive up prices and choke the industry. See below.
Many reactor designs have derived from US designs, so this bizarre complexity has had a global impact. The industry co-operated with the drive to make things as complex as possible, partly because suppliers loved whacking a nuclear surcharge on every item they supplied for a reactor, and partly because much of it happened before cancer epidemiology had established the much greater relative carcinogenicity of lifestyle choices. More on the US nuclear regulatory debacle later.
What are today’s actual prices for the stuff on the right? The International Energy Agency (IEA)’s estimate (in the Net Zero by 2050 (NZ2050) report) for combined-cycle gas turbine (CCGT)s (the most efficient gas turbines) is about $US1,000/kW in the US and about half that in China.
What’s the theoretical minimum price of the stuff on the left?
The Chinese have a reactor called the Happy200. It’s built for heat and not electricity. So it doesn’t have all the turbines and so on.
Can you guess the price?
Is it $US11,200/kW? That’s the CSIRO Gencost (GENCOST) estimate for the cost of an SMR. Unsurprisingly, that’s not even in the ballpark, as they say in the US.
Is it $US5,000/kW? That’s the IEA estimate for US SMRs. Still not close.
What about $US2,800/kW? That’s the IEA estimate for reactors in China. Warmer but still a long way off.
Its estimated price is just $US700/kW. The building of the first reactor is expected to start this year and finish by 2024.
So how about building one and then just adding a gas turbine and generator at the IEA estimated cost of about $US1,000/kW? That would drop the minimum theoretical price down to just $US1,700/kW.
Like most brilliant ideas, the brilliance isn’t in the idea, but the execution i.e, making it work. The hard bit is to match the interface, the temperature and the flow rate of the steam moving from the reactor to the rest of the plant. It sounds easy, but the volume of water being moved in the left-hand image may be 5-20 tonnes per second; depending on the reactor size. So there’s rather a lot of steam moving about between the two sides. And there may be multiple SMR boilers feeding one generator, or the other way round in a large reactor. Remember above when I said there were “lots of pipes” … that wasn’t a joke!
The Chinese do indeed want to make reactors that you can use to replace the boiler in a coal plant, but it it has to run much hotter than the Happy200 which is designed for district heating, not feeding a gas turbine. The reactor that the Chinese have designed for this purpose is the HTR-PM reactor. Late last year, the Chinese connected the first of 18 of these HTR-PM reactors to the grid. It’s the first of its kind and has been used as a test bed to improve the production engineering, which will make the subsequent units cheaper and faster to build.
A US-designed reactor which is similarly intended to be used with off-the-shelf generator components is the Thorcon reactor. The Thorcon design is being finalised in Indonesia with plans to build them in Indonesian shipyards. Thorcon estimate that they can build complete reactors for about $US1,200/kW. The people behind Thorcon have a background in building supertankers, which are definitely larger and, some would say, more complex than nuclear reactors. This gives them a very different mindset to traditional nuclear people. They estimate that once mass production is achieved, a single shipyard will be able to produce 20 x 1 GW Thorcon reactors in a year.
Nuclear failures
The history of nuclear power is no different from other high-tech industries. It is littered with failures. It’s easy to focus on the failures and ignore the success. Nuclear power is the largest generator of clean electricity in both the EU and the US.
Nuclear power’s success can be easily measured, as is clear from my previous post on build rates. Neither wind nor solar, nor their combined output has come close to meeting those historical nuclear rollout speeds; not when they were attempted the first time in the 1970s and 1980s, and not this time. This is despite the phenomenal advantages of computer-aided design, artificial intelligence in logistics and every other modern mass production advantage.
Will modern nuclear engineers fail to match the achievements of those who came before them and worked with slide rules and primitive computing resources? Perhaps they’ll fall into the trap of caring more about glossy presentations and clever ideas than actually building stuff which works. We’ll see.
Regulatory oversight
Future nuclear construction costs will depend on how well the nuclear regulatory regime can be aligned with the realities of modern cancer epidemiology and the intricate understanding of how DNA repair mechanisms, only vaguely understood in the 1980s, have explained the relatively trivial carcinogenic impacts of the Chernobyl accident.
Full-sized reactors, generating 1000 MW or more, are like blast furnaces; they run at high temperatures, usually with steam and pressure, and always with plenty of highly radioactive material.
So they need a good regulatory system. But how good is good enough? As good as aircraft? As good as large ocean-going passenger liners? Or supertankers?
An LNG road tanker exploded in China in 2020, killing 19 and injuring 172. Can you imagine if an LNG supertanker exploded, in port? In 1989, a few years after Chernobyl, an explosion at a gas plant in the Soviet Union killed 575 and injured more than 800. It could be a joke on a comedy program … what’s the difference between a nuclear accident and a gas accident? People die in gas accidents.
Which industry is more tightly regulated – gas or nuclear?
US nuclear costs
Here’s a graph showing reactor construction duration times in the US from a paper by Jessica Lovering:

It’s pretty clear that US used to be able to build reactors and then, following the Three Mile Island partial meltdown in 1979, the wheels fell off. This accident demonstrated that containment buildings work; they turn potentially nasty industrial accidents into “merely” expensive ones.
Is it credible that engineers started dropping acid and became stupid after 1979? Did they forget how to do their jobs? No. Put simply, the US Nuclear Regulatory Commission went rogue. One of the hard things about regulators is to ensure they aren’t “captured” by the industries they regulate. But it can work the other way also. They can be captured by an activist opposition. Once this happens, throwing sand in the engine is pretty simple. Changing the rules during the build is a terrific trick. Make the builder do everything again. Require complex changes on the fly. For some history, see here. In more recent years, starting in 2005, the NRC Chairman was an explicitly anti-nuclear activist with no nuclear engineering background, no oncology background.
In short, the NRC has been broken for decades.
Consider, for example, reactors like Canada’s CANDU model, with 34 reactors built in seven countries. These reactors run on natural uranium (i.e.,don’t need enrichment) and can be refuelled while the reactor keeps operating. They aren’t approved by the US Nuclear Regulatory Commission (NRC) (aka the Nuclear Rejection Cave). The CANDU application was withdrawn after the Canadians realised the futility and expense of the process.
Here’s how the obstruction works for new designs. If you submit one, it is reviewed by an NRC team charging $300/hour/person. Then they submit questions, and review your answers at ... you guessed it ... $300/hour for everybody on the team. Rinse and repeat. This goes on for years; typically, until you walk away.
But you can’t think about reforming nuclear regulation without considering the grab-bag of claims about the risks that nuclear reactors pose.
What about terrorism and weapons?
Nuclear weapons and materials have been a convenient plot line for action genres for decades. Take them seriously and you’d be believing that many weapons are stolen annually and that bombs are easy to make with material from power reactors that is also readily stolen. The reality couldn’t be more different. Stealing spent fuel rods from a cooling pond, for example, is about as easy as stealing molten lead from a blast furnace.
And dirty bombs? Things that go bang and spread radioactive material over a large area. Perhaps one or more of the many deadly gas explosions that occur annually are actually dirty bombs, covered up, of course, by the FBI, MI5, MI6, CIA and NSA.
How would anybody know?
Radiation is so poor-a-carcinogen that you’d need very fancy statistical methods to detect any change in cancer rates from such an event. I’ll provide a reference below that goes into considerable detail on the mechanics.
In any case, terrorists have never been smart enough to build any weapon of any sophistication beyond simple explosives, nuclear or otherwise. The closest to a sophisticated weapon was the sarin chemical attacks which killed 14 people in Tokyo in 1995. Building things has never been a terrorist strong point. The terms “building” and “terrorism” are intrinsically contradictory. Terrorist bomb makers frequently blow themselves up.
Terrorists are about terror and can achieve their goals with simple bombs, hijacked trucks, their own trucks, machetes and so on.
Have terrorists ever built an atomic device of any kind? An enrichment facility to make bomb-grade material from power reactor-grade material? No and no. Rogue nations take years or decades to accomplish even rudimentary bombs and processes. Non-rogue nations similarly. The Hollywood fantasy of it being easy might sell tickets, but it’s pure bullshit.
John Mueller’s book “Atomic Obsession: Nuclear Alarmism from Hiroshima to Al-Qaeda” is a useful vaccine to kill or prevent viral memes about risks from nuclear terrorism. If you are infected, I’d recommend you read 10 pages a day, and make sure you finish the course!
Accident risks
I’ve written about accident risks in other posts, like this one.
But just briefly. Carcinogenic risks of even the worst nuclear “disaster” are trivial compared to lifestyle choices; nuclear power is safer than bacon is a good, and accurate, way to think about it.
There were 148,000 bowel cancer cases in Japan in 2020, up from about 20,000 in 1975.
What caused this surge? It’s truly massive, much like the lung cancer wave that follows about 20 years after the introduction of smoking.
The Japanese surge was caused by dietary change, mostly increased red and processed meat consumption, and it’s not hard to calculate the additional bowel cancer cases: about 2.7 million extra cancers since 1986 (the Chernobyl accident year). You can see a similar pattern in any country with a recent increase in red and processed meat consumption, e.g., South Korea and Thailand.
The bottom line? Nuclear reactors can kill and injure people and need good regulation, but they aren’t dangerous like aircraft or passenger ships or dams. Nor can nuclear accidents pose a health risk remotely matching those of alcohol, cigarettes, bacon, obesity or inactivity. So they need far less regulation than any of these. But instead, they have far more than all of them combined.
Regulatory bodies need to be reformed to reflect the actual safety of nuclear power and the weak carcinogenicity of radiation at plausible levels, not just the fears driven by obsolete science, long known to be wrong.
Who knows how far reactor build times and costs could drop with proper regulation?
END PART II Next post … Total system costs
Comments