Just why did South Australian Energy Minister Tom Koutsantonis want to de-moth a couple of moth-balled diesel generators?

Okay ... that's a picture of a diesel generator, but it's totally misleading. Here's a picture of one of the generators in question. Sorry, but all you can see is the switching yard and the three small towers on the left are exhausts of the actual 3xturbine set of this 63MW power station.
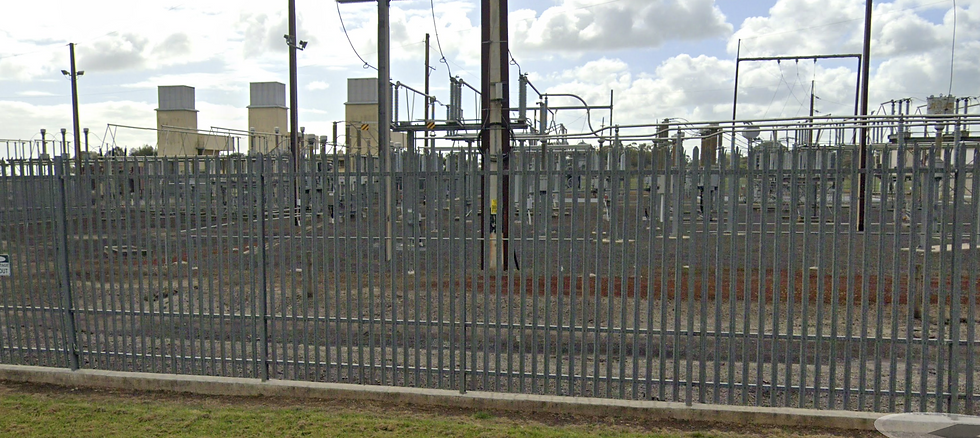
Koutsantonis stuck toothpicks under the fingernails of more than a few renewable-only energy advocates with his application to recommission a couple of decommissioned diesel generators to keep the lights on in SA, in the event that NSW and Victoria don’t have any excess juice to bail us out during any hot or cold windless nights, days, weeks or months.
Back in 2016, SA closed down our last 760 megawatt (MW) of coal generation. We traded it for 820 MW of transmission lines connecting us to Victorian coal. The trade was quickly followed by a statewide blackout (September 2016); ouch. So we installed four synchronous condensers to cover for the missing inertia in the grid.
Don’t sweat if you don’t understand that last sentence, nobody except the engineers understood it back in 2016, and nobody was listening to them until after the shit hit the fan. And even then, more than a few people blamed the flying shit rather than the rotating blades of the fan.
Synchronous condensers are massive spinning lumps of metal that provide inertia to replace that lost when you swap coal for wind and solar. They rotate at the same frequency as our AC current and resist change in the event that electricity demand changes rapidly; or you lose a generator suddenly. Theoretically, there are now alternatives to large spinning lumps of metal; but testing such innovations at scale is far from easy. Elon Musk may not mind his rockets crashing and burning, but crashing a grid isn’t the kind of headline that inspires politicians.
Aside from the condensers, Australian Energy Market Operator (AEMO) (our grid manager) also warned SA pretty forcefully (see Appendix B below for details), that we were still screwed without yet another transmission connection from the East. So work was begun on the $2.3 billion Project Energy Connect.
Having one transmission line fail is obviously a credible risk, but two? Clearly Minister Koutsantonis has been watching the chaos unfolding interstate and realises he should minimise our level of dependence. Having presided over the 2016 blackout, he’s not keen on an encore.
But diesels? Really? Is that the best he can do? Filthy toxic fumes; and is it even enough?
The two diesels in question will only generate a total of 138 MW and our peak power use during summer could be well over 3,300 MW.
SA’s record consumption was set back in 2010 at 3,397 MW and we hit 3,120 MW last year.
But what about all our renewables?
Renewables are irrelevant in energy planning
When the wind is blowing we can get close to 2,760 MW; which is normally more than 100 per cent of demand. But when it was 40 degrees last Thursday (4th Dec) at 4pm, the state’s wind farm output totalled just 63 MW.
The bottom line is that on a windless 40 degree evening in South Australia, all our wind farms and photovoltaic (PV) panels may well be irrelevant; AWOL. We may be 100 per cent reliant on gas, interconnectors, and yes, diesels.
How much gas power have we got? Currently, we have 11 generators with a maximum combined power output of 3,408 MW (assuming they can get the gas; more on that later). There is also another 450 MW of diesel, assuming we get that 138 MW back in service as Koutsantonis is planning. See Appendix C for a little more background on gas.
You always need to allow for generators breaking down or being unavailable. But it’s a different kind of risk. In smarter regions of the world, you pay them to be available and, as a result, they get planned maintenance done on time and are highly reliable when required; because the contracts fine the hell out of them if they aren’t.
The mechanisms for deciding exactly how much reserve capacity you need are complex. But clearly the situation is tight and Koutsantonis says that AEMO is warning of a 200 MW shortfall.
Let’s run through the options.
Build more wind and solar farms.
That won’t work. As explained above, it doesn’t matter how much wind and solar you have, because it can go AWOL for long and unpredictable periods.
Consider the following chart for the beginning of June this year. It gives the demand in South Australia and the sum total of all wind and solar output (including rooftop solar).

You can see that there are runs of nights with very little wind. If anyone wants to accuse me of cherry picking this particular period, then go ahead. Mea culpa. That’s how planning works. You find the hardest problem and see if you can solve it. Nobody gives a damn about the record level of solar power on the 5th day of whatever month. That’s the stuff of spin and headlines and not the stuff of responsible planning.
Just to drive home the point, here’s what would happen with twice our current number of wind and solar collectors:
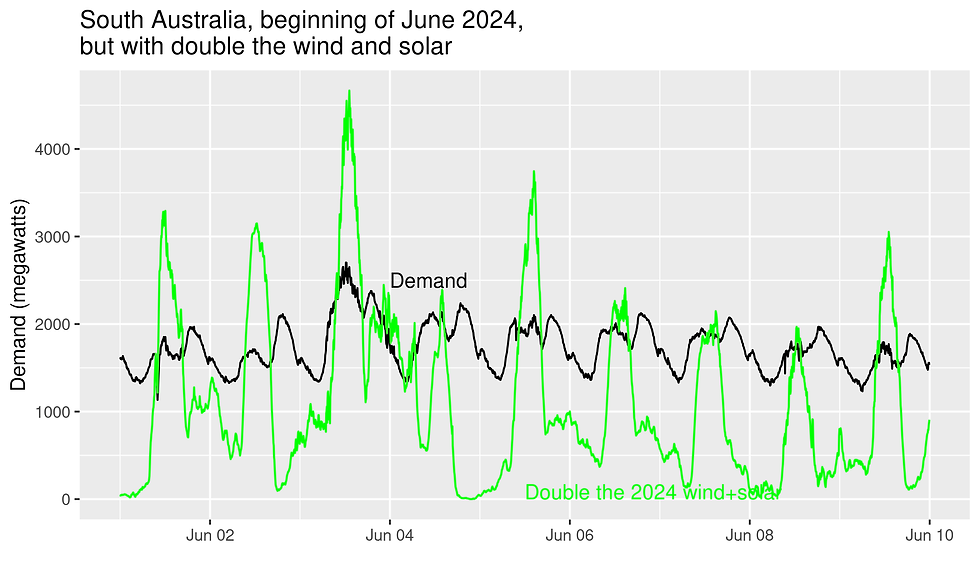
As you can see, twice bugger all is still bugger all, and there would still be sequences of days when answers aren’t blowing in the wind.
I could have extended this graph for the entire month, there were plenty of windless nights following this run.
Batteries.
Batteries are neither low carbon nor cheap. Especially if you need large banks which do nothing for most of the time.
Batteries are manufactured with mined materials which are processed in many energy intensive ways. The International Energy Agency (IEA) has an electric vehicle (EV) life-cycle emissions simulator and estimates the emissions during battery manufacture for an EV at 90 kg of CO₂eq per kWh. Grid batteries can have a lower carbon intensity, but that will depend on the technology. So the IEA figure gives us a ball park number of about 90,000 tonnes of CO₂eq per gigawatt-hour of battery energy storage capacity.
How many hours of battery storage would we need in SA to cover for windless nights?
Here’s a simulation with 11.5 gigawatt-hours of magic batteries connected with magic transmission lines.
Making 11.5 gigawatts of batteries will generate about a million tonnes of CO₂.
What are magic batteries and transmission? In this simulation, any excess electricity can always be stored in our batteries, regardless of where the excess and the batteries are located. In practice, the $2.3 billion price tag on the new 800 MW transmission line (Project Energy Connect) shows just how expensive it is to move electricity around, especially when you are talking about many gigawatts. For more on this topic, see the Appendix A.
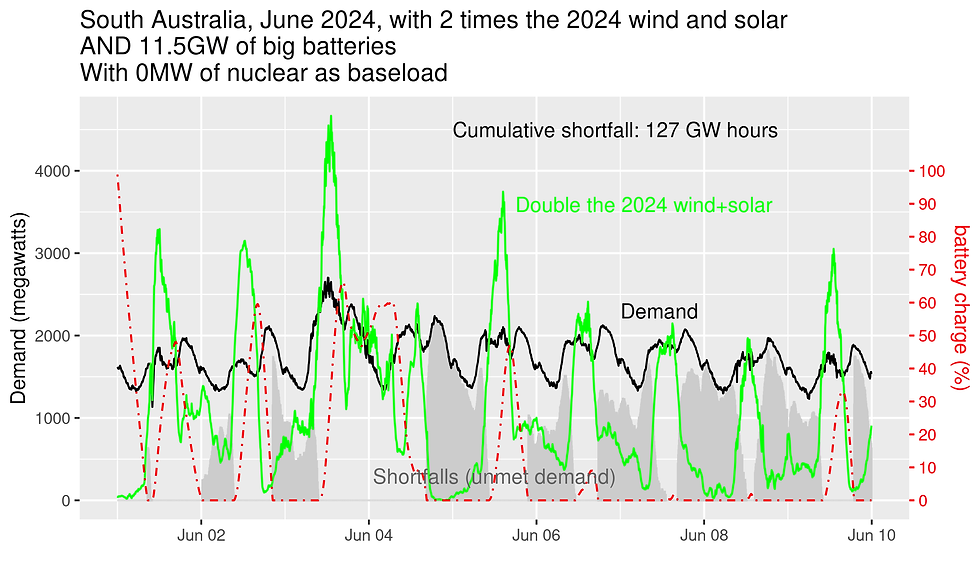
Let’s walk through this. The red line shows the battery status, starting at 100%, dropping rapidly, then rising to about 50% before being drained to zero. Late on June 3rd the battery is about 65% charged, but June 4 hits it hard and it doesn’t recover before the run of 4 still nights. The grey areas are unmet demand … meaning blackouts.
As you can see, even with 11.5 gigawatt-hour (GWh) of batteries, (about 23 times more than we currently have) the lights will go out and stay out, night after night after night.
The current price of 11.5 gigawatt-hours of Tesla Mega-Pack grid batteries is about $4.3 billion. That’s a considerable sum of money for something that will definitely fail multiple times in an average year.
Now you are starting to see how cheap a $2.3 billion interconnector is if there is something reliable on the other end of it.
Gas
Once you realise that batteries aren’t ultra-low carbon, like wind and solar, then it makes sense to compare gas with batteries on a variety of measures rather than simply saying “gas-bad, batteries-good”. Let’s assume a battery is good for 10 years, just to make the calculation simple. We can divide that million tonnes of CO₂ by 10 and think about how much gas can you burn to produce a similar amount (100,000 tonnes) of CO₂? Using a factor of 440 gms-co2/kwh, we get around 227 GWh per year. I haven’t included the whole of June or talked about how much energy those 11.5 GWh of batteries were producing, but over the entire month, they produced about 113 GWh while leaving a shortfall of 232 GWh. So if we used gas for the entire 345 GWh, we’d produce more CO₂ than making 11.5 GWh of batteries. If we used 15 or 20 years for the battery lifespan, then the carbon contest would swing more firmly towards batteries, but they still wouldn’t solve the problem.
The bottom line is that there is no low carbon solution to firming a renewable shortfall. The variability is simply too large. How do you reduce the variability? The simplest way is with a low carbon baseload source of power; hydro or nuclear. More on this later.
You could alternatively increase the overbuild of wind and solar to triple. That will give you more to charge an increased flock of batteries; but that will require more mining and more carbon.
And the cost is considerable. A 500 MW gas plant is about $500m (easy to remember, if well rounded!), plus the fuel to produce 345 GWh, about $33m. But the maximum shortfall isn’t just an energy problem but a power problem. The maximum power we needed was pretty much the maximum demand; a bit over 2 gigawatt (GW). So we’d need about $2.5 billion in gas generators; and they’d be sitting around doing nothing for most of most years; just like batteries.
So, despite needing a bunch of hardly used generators, a gas solution is cheaper than batteries which are both incredibly expensive and you simply can’t buy enough to solve the problem.
How many batteries would you need to be sure of keeping the lights on?
Unfortunately, my simple model can’t answer that, because it assumes magic batteries and a magic grid which can instantly store excess power and always get it back from the battery as required. Such a model can highlight what isn’t possible. If my magic model can’t cover the weather gaps, then it’s absolutely certain that a real world battery system of the same aggregate size will fail. But if a magic model succeeds, it doesn’t prove that a real world system with that capacity will succeed; because the constraints on a real world system are much stronger.
But I tested 20.2 GWh, that’s about $8 billion worth of batteries and it still won’t get us through the month without relying on interconnectors.
Let’s think about what we are doing here. We are trying to cover the worst case, no interconnectors and still weather. Clearly, that’s what Koutsantonis is trying to do. The probability of needing those diesels is tiny. Nobody really cares about having a few diesels hanging around that you’ll probably never use. On the other hand, who will invest in $8 billion worth of backup batteries that’ll hardly ever be used? And which would still leave you weather dependent if required.
Firm (clean) baseload (UPDATED)
The wind and solar marketing gurus have worked long and hard to convince people that “baseload” is an obsolete term. This section will convince you that it isn’t true.
Think about it. Add a couple of 600 megawatt (MW) nuclear reactors to the SA grid, in addition to our wind and solar, and suddenly those massive gaps you need to fill on windless nights shrink. Why 600 MW? That’s close to the maximum size a grid of the this size could handle. Back in 2012 and again in 2015, Ben Heard and James Brown recommended this as a decarbonisation solution. Koutsantonis wouldn’t have to be juggling with diesels if that report had been actioned.
A chart above showed that even over 11 gigawatt (GW) of batteries didn’t come close to handling the particular case of June 2024. I showed the first 10 days, but the whole of the month would have been plagued with trouble if the interconnectors were out of action for any reason.
Now lets show what happens when you add nuclear baseload to the system.
First I’ll repeat one of the charts above. I’ll call it the base case and it assumes 11.5 GW of storage and double the current wind and solar output.
Base case: 127 GWh shortfall
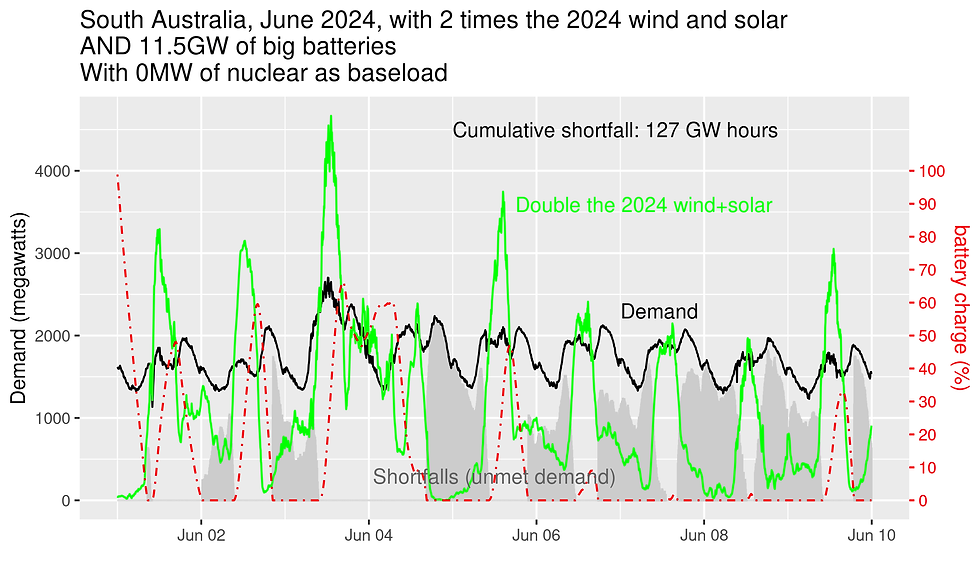
We've already seen this chart. You can see that even this massive battery bank is easily flattened, and once flat, needs rather more sunshine than this dull month provided.
Case 2: 25.4 GWh shortfall
Adding a single 600 MW reactor running as baseload slashes electricity shortfall from 127 GWh to 25.4 GWh.
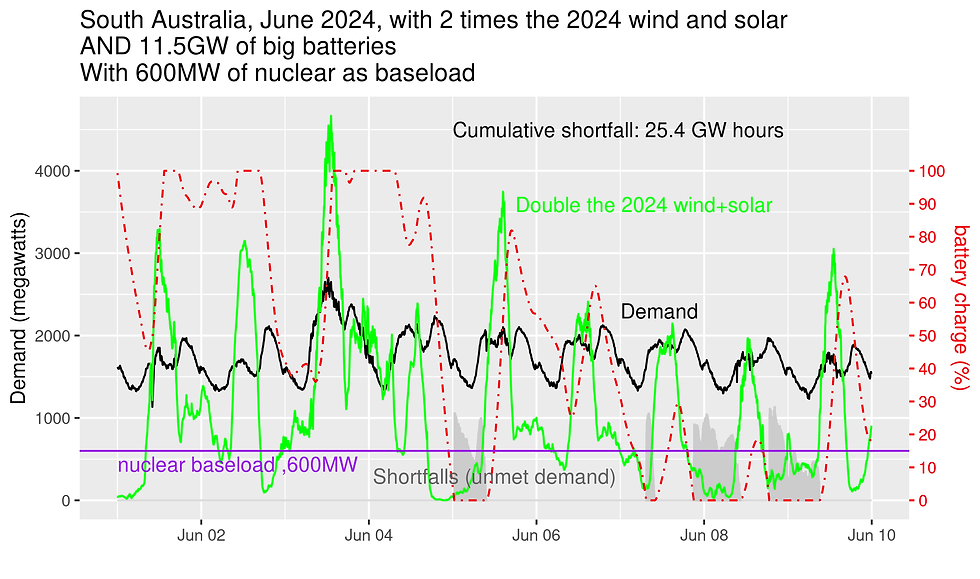
Case 3: Zero shortfall
Adding a second 600 MW reactor eliminates any shortfalls; assuming the various caveats I’ve given above about magic batteries. We can at least now start thinking about the far harder task of modelling real batteries at specific locations.
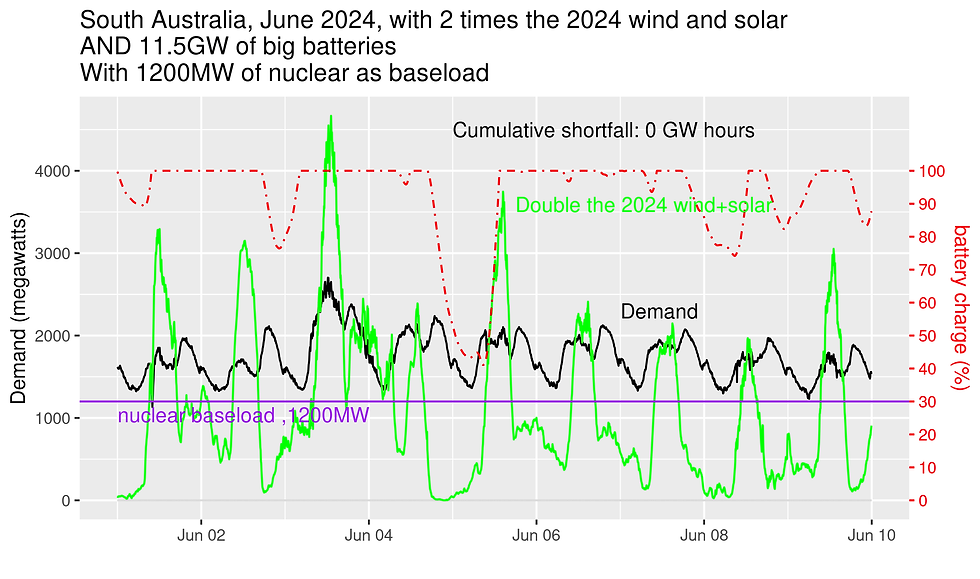
Case 4: 1.27 GWh shortfall
But what if we then halve the size of the battery bank? We don’t stay at zero shortfalls, but 1.27 GWh is pretty small.
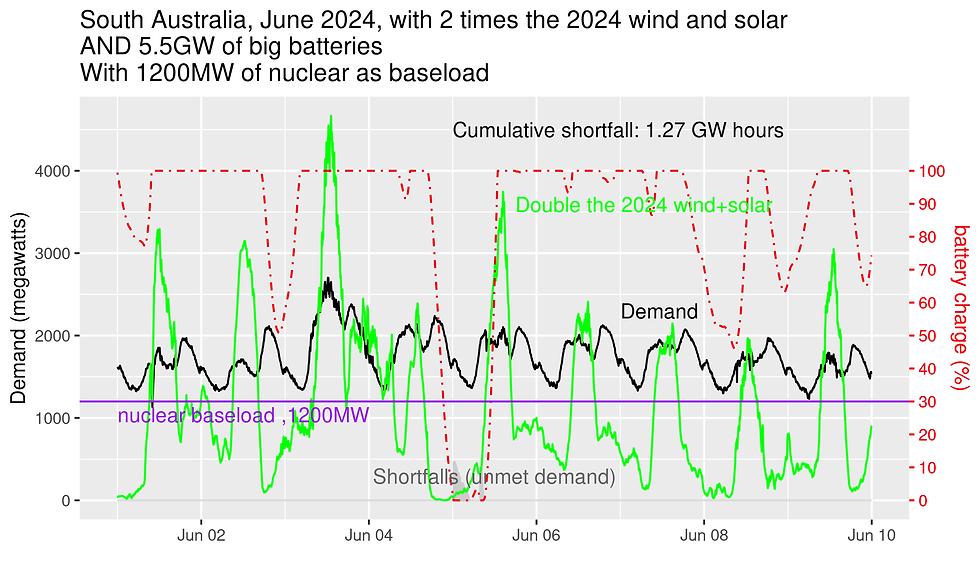
Case 5: 2.12 GWh shortfall
What if we didn’t double wind and solar? But just increase it by 40%?
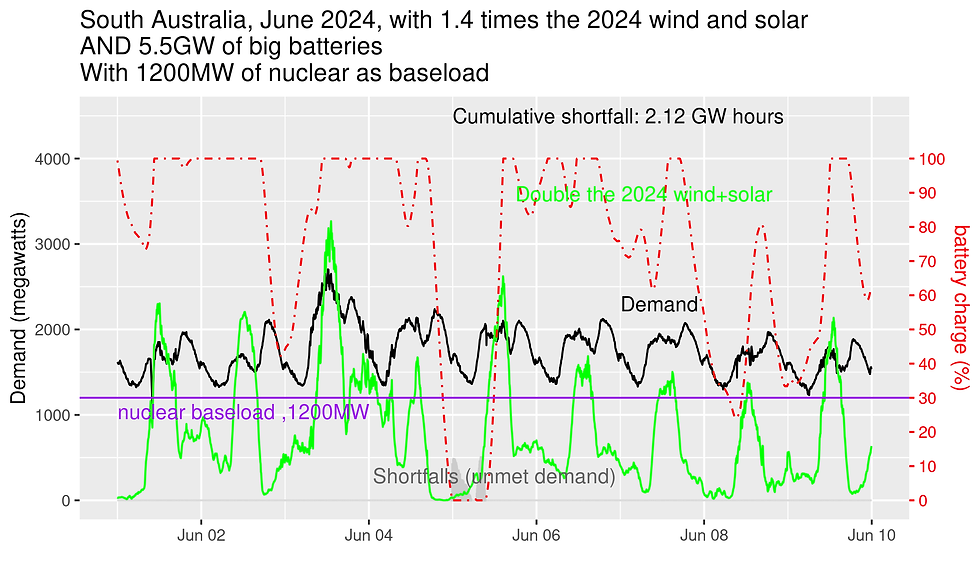
That’s remarkable. The 1.2 GW of baseload has allowed us to avoid 6 GWh of batteries plus a couple of GW of wind and solar while still delivering a very small shortfall.
This level of shortfall can be managed with small amounts of gas for a smaller carbon footprint than batteries. The caveat being that operating gas in this fashion is practical (see Appendix C below).
The US Department of Energy (DOE) released a report recently citing far more complete research showing the cost and carbon advantages of a system underpinned with firm baseload power. You need less of everything!
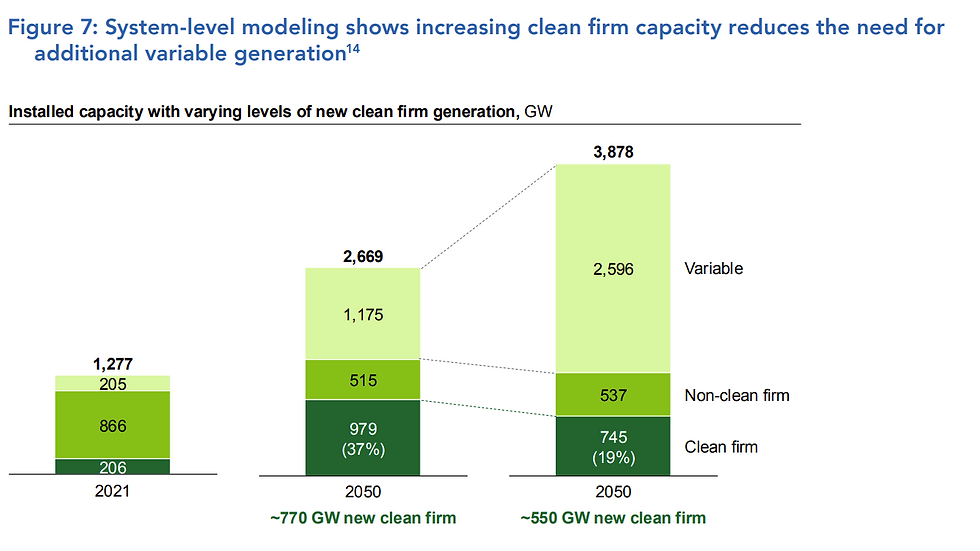
At this point you realise the pointlessness of the Commonwealth Scientific and Industrial Research Organisation (CSIRO)’s Gencost report. The cost of a grid isn’t about the LCOE of each type of generator. The interactions are critical to how much of everything you need. And the interactions flow right down to the transmission lines and the costliest part of the grid; the distribution.
Just a note about Canada’s CANDU reactors. They are typically a bit smaller than many large reactors, between 500 and 900 MW. They can remain on-line while refuelling; pretty amazing. That said, you would plan reactor maintenance for the times of the year with the lowest risk of inclement weather, and, obviously always keep one reactor running.
Having a reliable baseload segment removes multiple stresses from an electricity system and makes everything much cheaper and easier; which is exactly what the US DOE concluded.
Interconnectors aren’t baseload
The interconnectors are fine if we assume they will always be available. When Project Energy Connect is complete we will have two; which would hopefully mean at least one will always be available. The major problem is that our eastern states are making the same dumb decisions that SA has made; building weather dependent systems without any practical or financially feasible means of backup. Koutsantonis is right to be concerned. In any event, we can’t use the interconnectors as baseload. Having baseload massively reduces the intermittency holes you need to plug. More on this later.
The Government’s job
It's the Government’s job to supply electricity, on demand, at the best price. It’s also the Government’s job to ensure Australia does its bit in eliminating greenhouse gas emissions. All over the world, in every community, be it a village of 150 or a city of 5 million, there is a temptation to think “our little bit won’t make a difference”. Which is true, if everybody else does their bit. But if everybody wants to bludge on their responsibilities then we are all collectively buggered. Our Government, like others, has made international commitments, and should stick to them. But building a system which won't work isn't sticking to them.
Nobody can oppose people reducing their electricity usage, but that isn’t a sensible decarbonisation option for the simple reason that you can’t do enough of it. Nor is it without risk. The frail and elderly can, and do, die for want of air-conditioning on hot days. Making them feel guilty for turning it on, if they have it, is despicable.
Predictably, renewable energy advocates responded to Koutsantonis' diesel announcement by saying we don’t have enough “demand side options”. Here’s what they reckon:
“The lack of demand side participation, and the focus on energy supplies, has been one of the big frustrations for many analysts, and particularly the proponents of energy efficiency and other demand side measures whose entreaties over the past two decades have largely fallen on deaf ears.”
Note the lack of numbers. They aren’t saying how many consumers they think can and should switch stuff off during energy inclement weather. They aren’t saying how much stuff they should switch off and who should switch it off.
They have been advocating a weather dependent system which can't be firmed up with anything other than a mining boom and supertankers full of batteries AND gas. Baseload nuclear works and has worked for decades. It has the lowest carbon footprint of any source of electricity, as well as being the most eco-friendly.
Appendix A: magic batteries and magic transmission lines
You need at least two numbers to describe a battery:
its maximum power. For grid-scale batteries this is in megawatts (MW) and
either its energy capacity or, equivalently, the length of time it can provide its maximum power.
Energy is measured in megawatt-hours. So if you have a 500 MW that can provide this 500 MW for 2-hours, then it has an energy storage capacity of 1,000 MWh (notice the ‘h’ that has been added; denoting megaWatt-hours).
A real battery has a maximum power rating. You can usually run a battery it at half power for twice as long; but you can’t run it at double the power for half as long; because the maximum is what it says it is.
Magic batteries don’t exist but can be useful to prove what you cannot do. You only need 1 number to define a magic battery; its energy capacity. If 11.5 GWh of magic batteries isn’t enough to fill intermittency gaps, then 11.5 GWh of real batteries can’t do it either; no matter where you put them or how you size them.
On the other hand, if 11.5 GWh of magic batteries is enough to cover windless nights, it says nothing about what you can do with real batteries. Typically if you want to know if something is possible, you start by checking if it is impossible with magic batteries. If it is impossible, you can stop. If it is possible, then you need to do the much harder job of working out if there is a real configuration of real batteries and real transmission lines that solves your problem.
Appendix B: AEMO’s warning to South Australia back in 2020
Here’s what AEMO told the Government in writing; I imagine the verbal briefing was more blunt:
“If EnergyConnect does not proceed, extensive further measures (beyond those outlined in this report) will be required to address identified system security risks. Potential further measures could include commissioning significant utility-scale storage to provide FFR [fast frequency response], retrofit of a large number of distributed PV systems to improve disturbance ride-through capabilities, resistor banks for managing excess distributed generation, and possibly a moratorium on new distributed PV connections.”
Appendix C: Gas and practicalities
Over the past 27 years, with the anti-nuclear movement blocking the only real competition fossil fuels have ever had, Australia has built about 42,000 kms of natural gas pipelines. I’m not talking about the pipes into houses, but the big fat pipes which bring gas from gas-fields to cities.
We can, of course, get rid of gas. We just stop production and mothball the pipelines. All we need is an alternative.
But can we have gas “on-standby”? Just keep it around for backup purposes?
This is a political concept; not an engineering one. If you want to get gas out of a pipe at one end you need to be pushing it in at the other; to provide the pressure. That’s why gas is sold in bulk in contracts covering years.
If SA, or Victoria or NSW don’t produce enough gas, then they will have to import it as Liquified Natural Gas (LNG), perhaps from Qld or WA, or from overseas. Because LNG is liquefied (the clue is in the name), it is expensive. To liquefy it you have to cool it to -165 °C. About 10% of your gas will be used to provide the energy to do the cooling. So if you are really aiming for high priced electricity in Australia, you’ll run down the gas supply chain and rely on imported LNG to fill the gap.
Nuclear, on the other hand, is quite different. You can store a few years of fuel on-site with no problems at all. You can ramp the reactor up or down. People like to operate reactors continuously at full output, because that makes for the cheapest electricity, but all modern reactors can automatically match their output to the demand (this is called load-following). They can’t respond as fast as a gas turbine or a battery, but they can match reasonably changes in power requirements.
I did not have "recommission 138 MW of diesel" on my clean energy transition bingo card. Could they be planning to run them on biodiesel (so they get the "renewable" badge AND the emissions)?